Our lab has four major research instruments which enable us to contribute to a large variety of condensed matter research: a ultra high-vacuum evaporation chamber, a closed-cycle LHe cryostat, a full-field Kerr microscope, and a magneto-optical Kerr effect setup enabling magnetometry and electronic transport measurements.
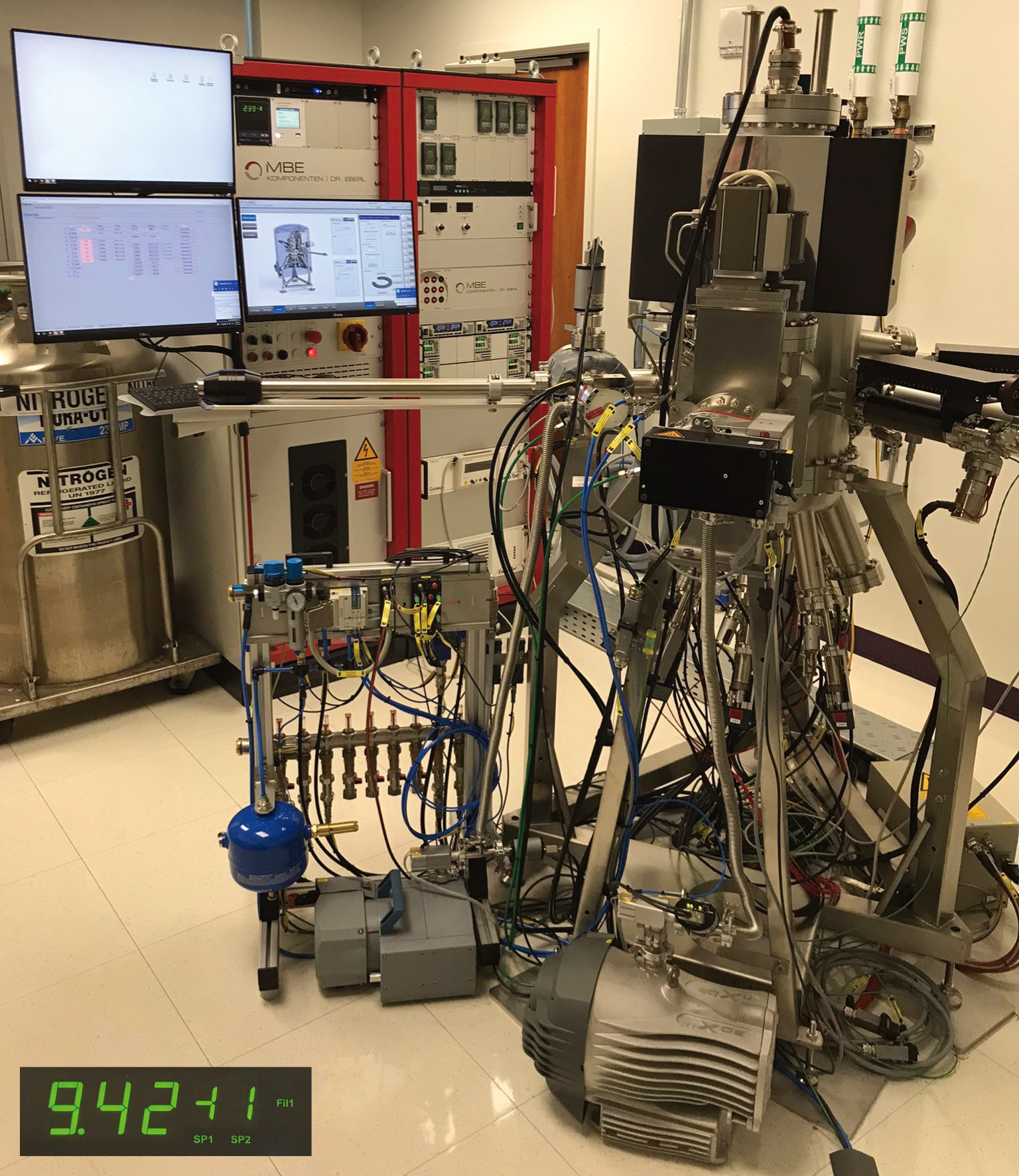
Molecular beam epitaxy evaporation chamber.
The ultra high-vacuum evaporation chamber (MBE Komponenten) is designed to synthesize amorphous, nano granular, and epitaxial films consisting of transition metals, metalloids, and/or rare-earth elements at variable substrate temperatures down to 100 K. It is equipped with effusion cells and an electron beam evaporator ensuring stable deposition rates for homogeneous and heterogeneous synthesis. Epitaxial film growth can be directly monitored with a RHEED detector.
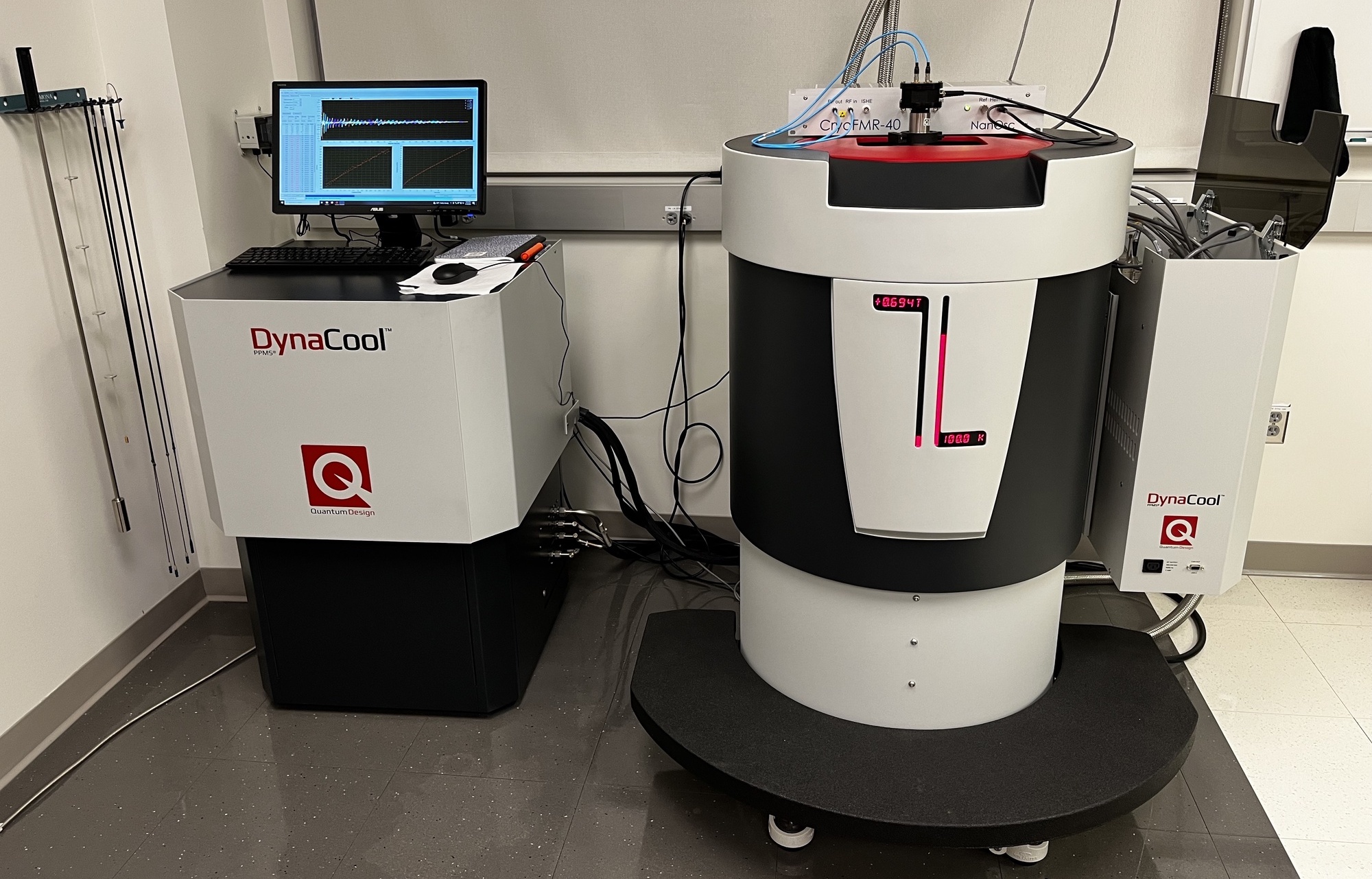
Quantum Design DynaCool Physical Properties Measurement System.
The magnetic films are characterized using our closed-cycle LHe cryostat (Quantum Design DynaCool Physical Properties Measurement System; 9 T, 1.7 K) to probe magnetic hysteresis loops (saturation magnetization, magnetic anisotropy, and transition temperatures), AC magnetic susceptibility (transition temperatures), magneto-transport properties (ordinary, anomalous and topological Hall effects, and AC and DC resistivity), and ferromagnetic resonance spectroscopy (saturation magnetization, magnetic anisotropy, Lande factor). Magnetometry and transport measurements can be performed at pressures up to a few GPa.
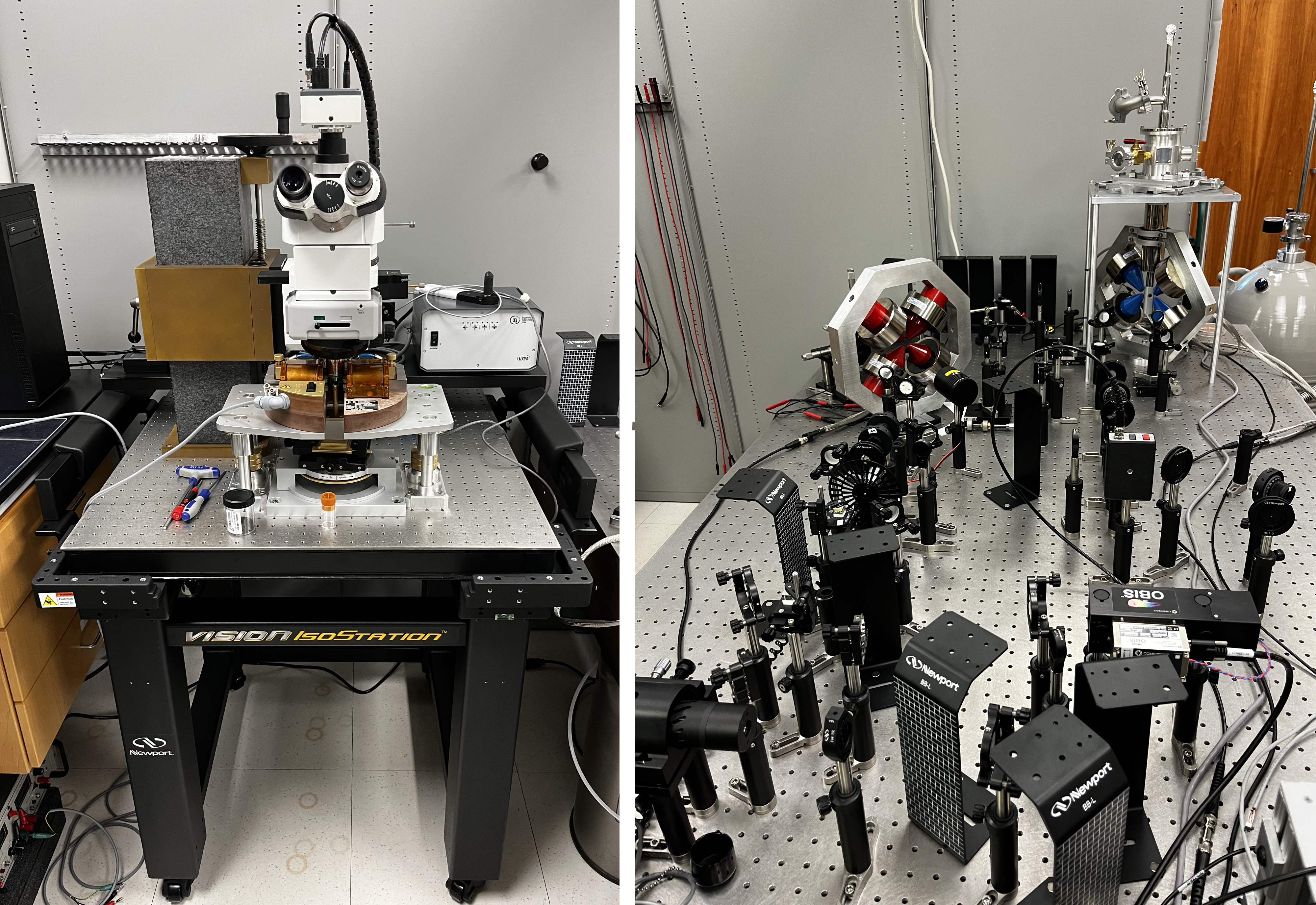
Magneto-optical Kerr effect microscopy and magnetometry.
Additionally, we have a full-field Kerr microscope (Evico Magnetics) and two magneto-optical Kerr effect magnetometers (home-built) for local surface-sensitive measurements of soft- and hard-magnetic materials, including films, crystals, nano structures, and structured liquids. The home-built setups are fully controlled by PyVISA/SCPI using a PyQt5 graphical user interface on OpenSUSE and use either an optical chopper (2 kHz) and dual-phase lock-in amplification or a photoelastic modulator. Both red and blue laser light can be used for probing metals and insulators and two quadrupole electromagnets for room-temperature and cryogenic temperature measurements (hysteresis, first-order reversal curves, magnetization relaxation, temperature dependence). It provides a playground for students to advance both experimental and hardware programming skills.
A high-performance desktop computer (Ubuntu) powered by 48 CPUs (500 GB DDR4 RAM) and one 48 GB RTX 8000 GPU and one 24 GB GTX 3090 Ti GPU facilitates numerical modeling of magnetic systems using open-source micromagnetic simulation frameworks (Nmag, Boris Spintronics, Vampire, and Spirit), iterative reconstruction of electron phases, and advanced data analysis. Additional computing power is provided by UNL's Holland Computing Center.
The Lab located at JH 341 is part of the department of Physics and Astronomy's Condensed Matter and Materials Physics group and works in conjunction with the Nebraska Center for Materials and Nanoscience (NCMN). NCMN is a central user facility adjacent to the Physics building, which offers nanofabrication, synthesis, and characterization capabilities essential to our research. We are further frequent users of large-scale user facilities of the Department of Energy, including synchrotron radiation facilities, electron microscopy centers, and nanofabrication facilities.
Our resources are used for both fundamental and applied research, and outreach and educational purposes.